The Life of an EV Battery From Cradle to Grave — and Why Recycling Is Still So Hard
Big batteries are fast becoming the norm for electric cars. But the lifecycle of one is still a convoluted, opaque process.
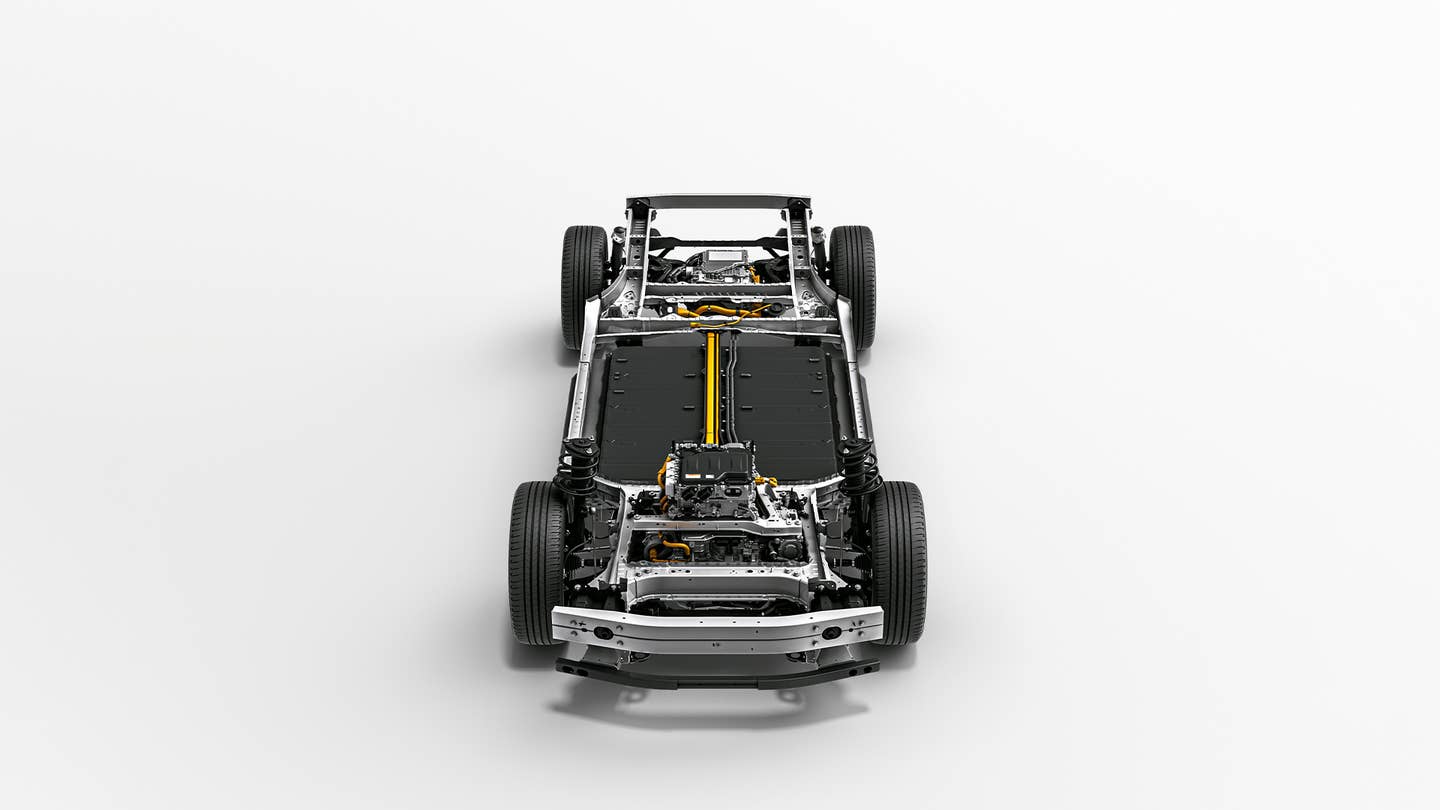
The move to battery-powered transportation is happening fast. If you had predicted 10 years ago that every automaker would have an ambitious plan to develop a full-electric lineup of cars, well, you'd probably be pretty rich right now. But a decade is a long time, and a perfect storm of factors—environmental, political, economic, and social—combined to bring about the most transformative moment the auto industry has seen since the Model T started rolling off Henry Ford's assembly line over a century ago. Talk of electric vehicles has been simmering for a long time. Now, it's at full boil.
As with any moment of great change and upheaval, though, there's no shortage of chaos to sort through. EVs are more prominent than ever, mass adoption seems just around the corner, and the promise of zero tailpipe emissions is presented as a golden opportunity for humanity. All true to some degree, but so is the fact that the whether or not electric cars take a big enough bite out of climate change will come down to one thing: the batteries. Or more specifically, how we design, build, manage and recycle that critical component, processes which are far less transparent (and far more important) that any number of splashy announcements about manufacturing partnerships or plans to launch 25 new EVs by 2030.
Like fossil fuels, the key ingredients going into today's batteries are also finite resources. It's obvious that developing ways to scavenge whatever can be reused from old batteries to build new ones will be critical in the years ahead, though doing so will require a level of cross-industry standardization we've yet to see on this front, and not all experts believe that's even in the cards. So today, we're taking a look at the life of an electric car battery from cradle to grave in 2022, from material sourcing to manufacturing to end-of-life recycling (or lack thereof), and what the current state of things means for what's next.
Battery Basics
In order to supply power to the one or more electric motors that power the wheels of an EV, there needs to be—you guessed it—electricity. Electricity itself can be generated in a number of different ways—burning fossil fuels, recovering kinetic energy, or renewable resources like the sun or wind. Nuclear power is technically on the table too, though we've written before about why that dream is likely forever beyond our reach.
Of course, the reason why we've settled on big batteries is because we need a method to densely store energy, and the principle of a battery-powered electric car isn't far off from that of an internal combustion vehicle with a gas tank. After all, gasoline is really just another medium to store and transport energy. Only instead of releasing that energy through controlled explosions under the hood, a battery converts stored chemical energy directly into electrical energy.
Electric cars aren’t new by any means. The first experimental electric vehicles were built all the way back in the 1830s (yes, really), powered by single-use batteries. By 1890, a Scottish chemist living in Iowa named William Morrison developed the first EV, a converted horse carriage powered by rechargeable batteries, technology that emerged by the mid-19th century. Historians do not agree on the chemical makeup of the batteries used in Morrison’s vehicle; some believe they were lead-acid, which had been invented just several decades prior by a French physicist named Gaston Planté. Regardless, the batteries gave Morrison’s carriage a range of about 50 miles with a recharge time of 10 hours. Not exactly ideal in a time when most homes in America still didn't have electricity.
The early 20th century saw a brief golden age of pre-war electric cars—after all, electricity was clean, bright, and new compared to the dirty business of burning coal and oil—and even competing steam-powered vehicles had a moment. There are a number of unsavory reasons why internal combustion still emerged as the most viable propulsion tech for vehicles by the 1920s, but on the practical side, high on the list was that it's an energy-dense substance that's relatively quick and easy to get into people's cars.
In 1996, responding to legislation in California that would've required every automaker to offer a zero-emissions car, General Motors wowed the world when it built the first modern, production-ready electric car by a veteran automaker in nearly a century: the GM EV1. The EV1 was initially produced with heavy lead-acid batteries, though GM did equip the second-generation EV1 with more modern nickel-metal hydride (NiMH) chemistry. The EV1 would go on to become one of the most controversially discontinued cars of all time, and the regulations that spurred its development were relaxed in favor of hybrids by the end of the 1990s, but it also proved to be a tipping point for EVs—Toyota, Ford, and other automakers also jumped with the Rav4 and Ranger EVs.
Momentum for electric cars was building, however slowly. Then came Tesla.
Many people know Elon Musk as the person behind Tesla, but the real face behind the company’s technical innovations was its former Chief Technical Officer, JB Straubel. In 2008, with the help of Musk’s investment, Tesla launched the first-generation Tesla Roadster with a new lithium-ion battery pack.

Led by Saraubel, the engineering team decided to build packs with many thousands of small cells rather than several large cells. This gave the team about seven times the surface area to dissipate heat, which helped immensely since the engineers also designed a way to cool the packs with liquid rather than just with the passing air. This innovation led to a car with an EPA-certified range of 244 miles: more than double that of the NiMH-equipped EV1, and nearly five times as much as those powered by the original lead-acid packs. In short, the modern electric car battery was born.
Inside an EV Battery
Most BEVs today use lithium-ion batteries to store energy. Though the chemical makeup of the battery can differ from manufacturer to manufacturer, the building blocks are relatively the same: an anode, cathode, and electrolyte. It's less important to understand the why behind the science for our purposes today; what matters is that electricity is discharged from the battery cell as the lithium ions travel through the electrolyte from the anode to the cathode (and the reverse when charging).
Like Straubel discovered, EV batteries work best with many small cells packaged into a usable form factor—whether that be in cylindrical cells like most Teslas (excluding LFP-equipped models), or prismatic (pouch) cells like those inside of the Hummer EV or Hyundai Ioniq 5. All of those cells require a substantial amount of lithium; some experts estimate around eight kilograms per vehicle, or about 17.6 pounds. For context, the average iPhone reportedly has less than one gram of lithium inside.
So where does it all come from? The majority of the world’s lithium comes from China, Australia, Chile and Argentina. Lithium is obtained through either open-pit mining or by being extracted from rich brine with a reagent (like sodium carbonate) and then dried until it forms lithium carbonate.
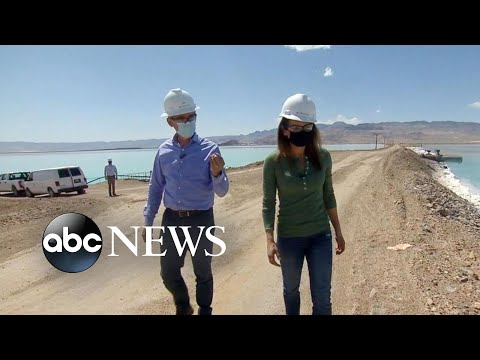
The dried lithium carbonate is then sent to processing plants where it is turned into cathode-active material. During this process, the active material is uniformly mixed into a slurry containing conductive carbon and a polymer binder, which makes it suitable to be used during the actual manufacturing process of the battery cell.
Other materials like cobalt or nickel are used in cathode production as well. It’s worth pointing out that this is one of the largest points of contention in the battery-building process, as cobalt mining has been a huge humanitarian issue fueled by exploitative conditions and child labor. Most companies have pledged to ethically source materials for battery production or have moved away from cobalt as a whole due to sustainability concerns. Thing is, there's not a lot of ethically-sourced cobalt floating around the world's supply chains.
Next, the cells must be assembled. While the fundamental workings of a battery are the same, the form factor used by automakers may differ. For example, cylindrical cells will have the components wound and encased into a metal cell, whereas pouch cells will have the components layered or “sandwiched” inside.

The cells are then placed inside an automaker’s proprietary packaging. This step, in particular, can vary depending on the manufacturer, though most companies will build those individual modules that can then be modularly assembled into packs before being installed in the vehicle.
“I don't think the box you put it in [matters as much]," GM's Director of Electrification Strategy Tim Grewe told The Drive. "A lot of people make a big deal about the box to hold the electrodes, [but] I think it has to do with the electrode characteristics, and how [uniformly] you can treat the electrode."
Grewe went on, “And whether you put the electrode in a can or a pouch—you know, truly the pouch has some very clear cost advantages to it—everything will coexist in the industry. It has a lot to do with your vehicles and your portfolio and how you integrate it into the vehicle as well.”
From there, the battery is slapped under an electric vehicle (another major oversimplification, of course) where finally it can be driven off a showroom floor and parked in your driveway.
Reduce, Reuse, Recycle
After a battery serves out its useful life—the length of which depends heavily on the number of charge cycles it's experienced, environmental conditions, and its daily use—it then needs to go through a disposal process.
This brings us to one of the most common points made by EV skeptics: pollution. It’s no secret that batteries are an absolute environmental disaster to produce—a vehicle with a range of 250 miles is estimated to produce 68 percent higher manufacturing emissions when compared to building a gasoline-powered vehicle. While this doesn’t account for tailpipe emissions produced during a combustion vehicle's service life, it certainly speaks volumes about the manufacturing impact of a BEV.
And what happens after a pack reaches the end of its usable life? Do all those cells just end up in a landfill somewhere? Not exactly, though not far off either. The industry is still trying to figure out the best way to reclaim old batteries to reduce environmental impact and avoid simply running out of lithium altogether, but the process is far from ideal.
The obvious answer is systematic recycling. Experts believe that around 1.6 million metric tons of battery packs will be ripe for recycling by 2030, and materials reclaimed from used, orphaned, or otherwise scrap batteries can be used in the manufacturing of fresh units. Rather than discarding the old cells and reaching back into the earth for more minerals, we can pull those precious resources out of what's already been made, the same way recyclers pull gold and silver and platinum out of old electronics. Cars have been using lead-acid batteries forever and we've got a pretty good system for recycling those, no matter what Google tells you. Why can't the same be true for these larger lithium-ion packs?
As Grewe mentioned earlier, a manufacturer will package batteries differently based on its exact business needs, which is the first hurdle in standardizing the process for everyone. For example, disassembling a pouch-cell battery versus a cylindrical-cell battery is a completely different process. Grewe also told me that the auto industry wants to enable the recycling industry to innovate in parallel—but is that achievable if battery cells aren’t uniform or easily reclaimable from one automaker to the next? No matter the form of recycling, batteries still need to be disassembled in order to be recycled. And the need to reuse something can drive product decisions; recycling alone is why many 12-volt car batteries share a very similar form factor today.
Regardless, after an electric car battery is disassembled, its internal components can be shredded and sorted, resulting in the rather sinister sounding clump of materials known as "black mass." Within the black mass is a wealth of valuable materials already mined from the earth: carbon, graphite, cobalt, nickel, manganese, and lithium to name just a few. But there is also plastic, steel, aluminum, and other containments that need to be removed. So the black mass then needs to undergo additional steps to extract meaningful materials, typically through either pyrometallurgy ("pyro") or hydrometallurgy ("hydro").
Pyro reclaiming uses extreme levels of heat (around 1,500 degrees Celsius) to reclaim materials through smelting. It does a great job at recovering cobalt, copper, and nickel. It also burns off graphite and any solvents, but that contributes to the process' rather high emissions output. It also doesn't do a great job at recovering lithium, as much of it is turned into slag (a waste byproduct) during the process, which isn't really financially viable to be turned back into usable material.
Hydro reclaiming is another option many recycling firms are moving toward. Rather than smelting, hydrometallurgy uses aqueous solutions to extract valuable materials from the black mass, which means a lot less energy wasted since the heating requirement is significantly reduced. Like pyro, it offers great cobalt, copper, and nickel recovery, but it also provides an option to efficiently recycle lithium, graphite, and manganese. Of course, there are drawbacks in its current state, the largest two being a significant amount of waste byproducts and a high startup cost.
The wildcard here is that so far, batteries are lasting longer and outperforming what most analysts and manufacturers have expected at this stage. Potentially good news for your wallet, but bad news for recycling innovations.
“Batteries are lasting too long,” Grewe said. “It's going to be one of those frustrating, good news stories, where there's not a large recycling market because the batteries are lasting longer than anybody expected.”
But battery longevity could also lead to a new view of car buying for the next driving generation. Tesla's Straubel, who started a battery recycling venture called Redwood Materials, believes that it's less likely that EV owners will replace aging batteries versus replacing a complete vehicle.
“It’s a subjective thing depending on what [a person's] goal is for the car, but I think it’s going to easily be 15 years [of ownership] in most cases,” Straubel said at an energy conference earlier this year. “I think battery life will probably track the life of the vehicle life. Personally, I think it’s less likely that people will place a new battery in an old car.”
What Straubel is describing kind of mimics the behavior that many ICE owners have now. When the average vehicle owner pops an engine, it's often cost-prohibitive for them to replace the car's motor, so the vehicle is often sent to a scrap facility or traded in (essentially for the cost of disposal) towards a new vehicle. With the replacement cost of a new battery pack nearing half the cost of a new vehicle, it's likely to think that the majority of owners not underwater on their loans will likely echo that behavior, similar to Straubel's predictions. Also not great news for keeping recycling near the top of the industry's priority list.
Charging Into the Future
Battery-electric vehicles are coming to a dealer lot near you if they're not there already, there's no denying that. The tech is still in its infancy in terms of mass adoption, and the way we think about building and maintaining batteries today is probably not how we'll think about them in 2032. Hardware and software may have problems, batteries may degrade differently than anticipated, and the charging network will take time to catch up. But perhaps the biggest unknown is how fast technology will change. Automakers like Honda and Toyota are already heavily investing in solid-state battery development, which could offer significantly faster charging times versus today's lithium-ion cells. Will that immediately take over once commercially viable?
According to GM's Grewe, not quite. “Lithium-ion and solid-state batteries will all coexist in the transformation because there is no clear winner yet,” he said. “I think it's going to be very healthy competition where you'll see it coexist in the industry for different reasons. And then it will converge on the lowest cost, best tech in the long run.”
What is certain is that automakers will be under some pressure to produce quality components when it comes to batteries. The California Air Resources Board (CARB)—the same body whose zero-emissions requirement brought the GM EV1 into existence 25 years ago—has already drafted battery degradation limits that are set to be enacted by 2026 if adopted. If the requirements were published today as drafted, vehicles must maintain 80 percent of their original rated range for at least 15 years or 150,000 miles. Presently, Hyundai is pledging a 10-year 100,000-mile warranty on the Ioniq 5's battery. Tesla has a similar warranty with 8 years for all of its vehicles, and a mileage range of 100,000 to 150,000 miles depending on the model. The Hummer EV also has a 100,000-mile battery warranty, though Grewe has previously stated that he expects the back to last between 150,000 and 250,000 miles.
No one said breaking up with gasoline would be easy. But getting back together with batteries will present its own host of problems and complications that we'd do well to understand and sort out sooner than later, lest we end up back in the same kind of troubled relationship.
Got a tip or question for the author? Contact them directly: rob@thedrive.com
